Analysis of Conductive Layering by Electromagnetic (EM) Vertical Sounding
Introduction
Vertical soundings, where low-induction-number (LIN) EM measurements are made at a range of heights over a given location, provide a convenient means for identifying conductive layering in the earth. Where conductive layering is present, sounding data are amenable to the interpretation of the conductivity and thickness of a surficial layer, along with the conductivity of the underlying earth.
Sounding Technique
The sounding technique should seek to minimize positional, environmental and geological noise. One aspect of positional noise arises from misalignment (i.e. pitch and roll) of the EM array. Careful visual control is often sufficient although tilt sensors, as incorporated some LIN EM instruments, are more accurate.
A standard of non-conductive material helps to reduce height inaccuracy, the other aspect of positional noise. Figure 1 shows a standard made of a wooden dowel and base. The interval between marks for height on the dowel is 0.1m.
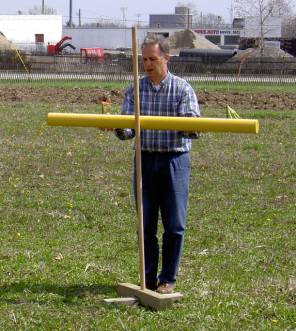
Figure 2 shows a standard with a PVC frame that enables measurements at heights up to 3.6m.
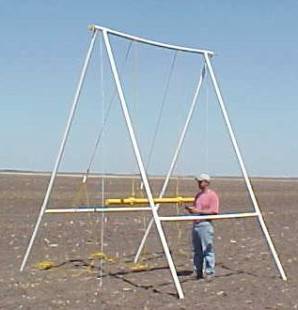
Where no standard is available, vertical soundings can be made with care by holding the instrument to various parts of the body with heights that are approximately known. For example, a sequence of measurements might be made with the instrument on the ground, then at the heights of the ankle, shin, knee, waist, neck and overhead.
Sources of environmental noise range from metallic and magnetic objects worn by the operator of the instrument, to nearby electrical devices, to distant electrical storms. For short arrays (e.g. 1 m), objects close to instrument tend to be more troublesome, whereas the proportion of noise due to distant sources tends to increase with array length.
The operator should remove rings, watches, glasses, etc., that would otherwise be near the instrument and to keep non-removable zippers, buckles, etc., as far away from the instrument as practical.
Electrical noise from nearby electrical devices, such as powerlines, often has a directional aspect; it may be possible to reduce such noise by testing several orientations of the instrument relative to the suspected source. The noise from atmospheric storms is generally directional, and a north-south orientation of the instrument is typically optimal.
Lateral inhomogeneity may cause geological noise, as the simple interpretation of vertical soundings described herein assumes that the earth is uniform or horizontally layered. A test for lateral inhomogeneity consists of comparing sets of measurements with the instrument in orthogonal orientations, e.g. north-south and east-west.
Sensitivity to Depth and Apparent Conductivity
At LIN, the sensitivity of an EM array to conductivity to a given depth is defined by the geometry of the array.
If the unit of depth is the length of the EM array, and the sensitivity to an infinite depth has a value of 1, the sensitivity function for the horizontal co-planar geometry (HCP) is:
h(d) = 1 – 1 / (4d2 + 1) 1/2
The sensitivity function for the perpendicular geometry (PRP) is:
p(d) = 2d / (4d2 + 1) 1/2
Where the material below the array consists of a given depth, a, of air with zero conductivity, over an earth with conductivity of e, the apparent conductivity will be the sum of the sensitivity to the air times the conductivity of the air, plus the sensitivity to the earth times the conductivity of the earth.
Thus, for the HCP array, the apparent conductivity will be:
ch = h(a) (0) + (1 – h(a)) e
= (1 – h(a)) e
Similarly, the apparent conductivity measured by the PRP array will be:
cp = (1 – p(a)) e
Figure 3 shows the functions 1 – h(a) and 1 – p(a), which represent the relative sensitivities of the HCP and PRP arrays to an earth beneath a depth, a, of air.
If the earth is reasonably homogeneous, the measurements from a vertical sounding will conform to the curves of Figure 3, with the common Y-intercept indicating the true conductivity of the earth.
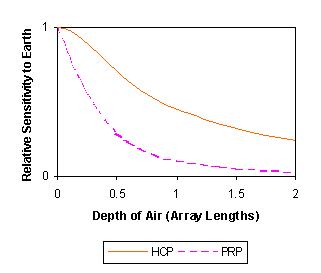
Figure 4 shows the measurements from the site pictured in Figure 1. As the measurements resemble closely the curves of Figure 3, the earth is essentially homogenous at this location, with a true conductivity of about 32 mS/m. The scale of the X-axis is proportional to that of Figure 3 as the sounding was made with the DUALEM-1S, which has an array length of about 1m.
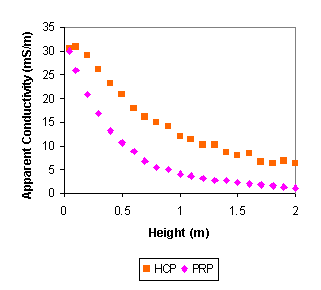
Where there is conductive layering in the earth, the apparent conductivities at low height will diverge, and the reductions in apparent conductivity with height will deviate from those for a homogenous earth. Figure 5 shows these characteristics in measurements made with the apparatus illustrated in Figure 2 (R. Eigenberg, pers. comm.).
For reference, Figure 5 also shows the curves for a homogeneous earth with 50-mS/m conductivity. The scale of the X-axis is proportional to those of Figures 3 and 4, as the measurements were made with a DUALEM-2, which has an array length of about 2 m.
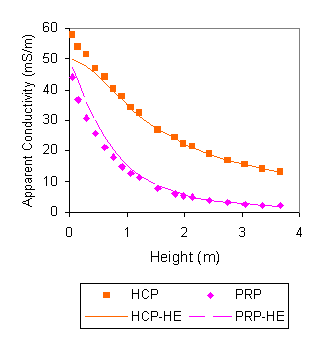
At low heights, the HCP array senses conductivity higher than that for a 50-mS/m earth, and the PRP array senses lower conductivities.
Qualitatively, the measurements indicate that the earth is less conductive near surface than at depth, as the sensitivity of the PRP array is shallow relative to that of the HCP array.
Figure 6 shows measurements made with a DUALEM-2 in the Mojave Desert, where loess has accumulated on top of a recent flow of basalt. The sounding was made by holding the instrument on the ground, and then at the height of various parts of the body. For reference, Figure 6 also shows the curves for a homogeneous earth with 40-mS/m conductivity.
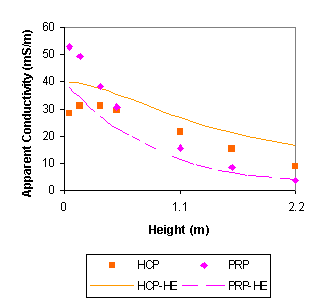
At low heights, the HCP array senses conductivity significantly lower than that for a 40-mS/m earth, and the PRP array senses significantly higher conductivities. In contrast with the previous example, the measurements indicate that the earth is more conductive near surface than at depth.
Layered-Earth Interpretation
The expressions for apparent conductivity can be expanded for an earth with multiple layers, but vertical soundings with LIN EM instruments usually are most suitable for characterizing an earth with surficial layer of thickness t and conductivity s, and underlying conductivity e.
Using again the sensitivity functions h(d) and p(d), along with depth of air a, the apparent conductivity measured over such an earth by the HCP array will be:
ch = (h(a + t) – h(a)) s + (1 – h(a + t)) e
The apparent conductivity measured by the PRP array will be:
cp = (p(a + t) – p(a)) s + (1 – p(a + t)) e
These equations can be programmed into a spreadsheet to predict the apparent conductivities that would be observed over an earth with given values for thickness and the two conductivities. The interpreter can then search for values of these parameters that minimize the discrepancy between measured and predicted values. The following examples demonstrate the results such interpretation.
Figure 7 shows again the measurements of the site depicted in Figure 3, along with curves for apparent conductivities that would be observed over an earth with a non-conductive surficial layer of 0.2-m thickness, and an underlying conductivity of 54 mS/m.
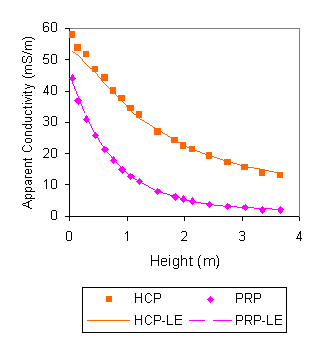
The measurements fit the curves of Figure 7 more closely than the curves of Figure 5, supporting the interpretation of a thin, resistive surficial layer.
Figure 8 shows again the measurements of Figure 6, along with curves for apparent conductivities that would be observed over an earth with a surficial layer of 1-m thickness and 70-mS/m conductivity, and an underlying conductivity of 1-mS/m.
The measurements show no systematic divergence from these curves, indicating that suitability of these layered-earth parameters of thickness and conductivity.
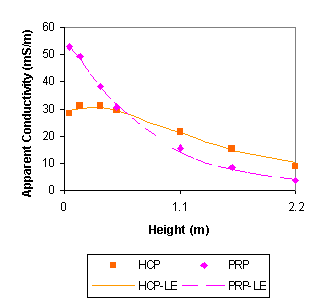
2-Layer Interpretation
An earth-model with two layers can provide useful insight into near-surface conditions in cases where accurate measurements of apparent conductivity show significant and systematic divergence from curves for one-layer models.
For example, Figure 9 shows the measurements of Figure 7, along with curves for apparent conductivities that would be observed over an earth with a non-conductive surficial layer 0.4-m thick, over a layer 4-m thick with 70-mS/m conductivity, over a remaining earth with 40-mS/m conductivity.
The curves of this model eliminate the systematic misfits seen in Figure 7. However, as these misfits are minor for the PRP measurements, and modest for the HCP measurements, this example shows a case where the 2-layer model is marginally more informative than the 1-layer model.
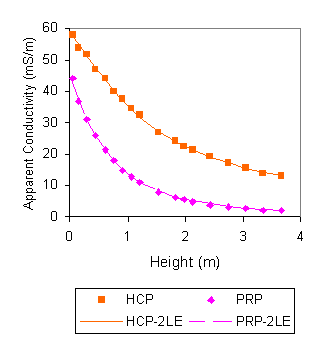
Conclusions
Vertical soundings with HCP and PRP arrays enable the convenient identification of conductive layering in the earth, as changes in apparent conductivity are distinctive where the surficial conductivity is either greater or less than the conductivity of the underlying earth.
Sounding data are also suitable for the quantitative interpretation of the thickness and conductivity of a surficial layer and the conductivity of the underlying earth.
Vertical soundings require careful technique and interpretation, which suggests that they might be used most effectively in conjunction with single-height surveys, where the nature of layering is of interest over large areas.
Dualem Products
DUALEM products are used by companies and universities on every continent. Our products fulfill a variety of use-cases in industry and academia.